STRETCH out your hand. Ever wonder what it’s made of? The skin masks flesh, blood and bone sure enough. But those tissues are made of molecules, which are made of atoms. And atoms are made of electrons, protons and neutrons. It’s only when we drill down to fundamental particles and energy that we reach bedrock.
Or do we? The history of physics certainly gives us pause. For more than 300 years we have been asking ourselves about the true nature of reality – what, ultimately, stuff is made of. Time and again, we have found another layer beneath what we thought was the lowest. What’s more, with each new depth we plumb, our old understanding of reality is swept aside.
Now we could be on the cusp of another revolution, thanks to efforts to reconcile our two most successful but incompatible theories of reality. Not particles, energy, space,time or anything else we might think of as fundamental truly is: instead, the essence of reality is a thing whose workings we’re only just beginning to grasp.
Every age has had its own list of reality’s basic elements. For the philosopher Democritus, everything was made of atoms. For Aristotle, it was earth, air, water and fire. In the late 19th century, all the talk was of the luminiferous ether, a medium which was thought to carry light.
For most of the past three centuries, however, Newton guided our thoughts on what all things are made of. He thought that reality had three elementary components: time, a cosmic clock ticking away in the background; particles with mass; and a space in which the particles moved, which he called the “sensorium of God”. With this trio, Newton constructed a framework for understanding the workings of the universe that was and continues to be astoundingly successful – as long as the particles are not travelling near the speed of light.
But even without that caveat, Newton’s work didn’t explain everything. Although it provided a description of gravity, the attractive force governing the movement of masses in space, it did not explain what it was. Soon there were other forces, like electromagnetism, which were similarly mysterious. Ever since, we have been embroiled in an ongoing renegotiation of the essential ingredients of reality (see diagram).
Today, the pressing issue is to unify quantum theory, our best description of the world at the smallest scales, with general relativity, Einstein’s masterly theory of gravity. When we try to describe black holes, dense cosmic objects that suck in everything including light, or the big bang, we require both these pillars of modern physics to work together. Then things stop making sense.
“Every tangible aspect of reality is likely to be an illusion”
The trouble is that quantum theory treats forces as coming in discrete chunks called quanta, but general relativity treats gravity as a smooth and continuous force. All efforts to harmonise things by quantising gravity have so far failed – but they have yielded some clues about what might underlie both theories.
The story of the latest revolution in our thinking begins in the late 1990s, when Juan Maldacena at the Institute of Advanced Studies in Princeton, New Jersey, was working on string theory, a proposed route to unifying things based on the idea that elementary particles emerge from the vibrations of one-dimensional “strings”. Maldacena showed that, for a given volume of space-time, a string theory describing gravity inside can be mathematically equivalent to a set of quantum equations, which describe the boundary of the volume but don’t include gravity. It all sounds rather technical, but this “Maldacena duality”, as it came to be known, hinted strongly at a connection between general relativity and quantum mechanics. That made it worth investigation.
It turned out to have an intriguing link to another concept in physics. In 1935, Einstein and his colleague Nathan Rosen had shown that two black holes could be connected by a peculiarity of space-time, called an Einstein-Rosen bridge – or colloquially, a wormhole. In 2001, Maldacena used his duality to show something extraordinary about wormholes: that they form because the quantum states of the two black holes, as seen from the outside, are entangled, which means they can spookily influence each other’s states over distance.
Then in 2009, Mark Van Raamsdonk at the University of British Columbia in Canada, began looking at what happens if you change the amount of entanglement between the black holes. He found that this controlled the width of the wormhole: increase it, and the wormhole expands, decrease it and you can snap the connection entirely (see diagram). It was as if entanglement, a quantum phenomenon, could create space-time, as described by general relativity (New Scientist, 7 November 2015, p 30).
Raamsdonk’s work led Maldacena and his colleague Leonard Susskind of Stanford University in California to an audacious hypothesis: that space-time in general is created by entanglement. It was a glimpse of a lower level of reality.
Make some space
But only a glimpse. The idea is not yet a fully formed theory, as became clear when Susskind started to mull it over in more detail in the context of a different quirk of space-time: single black holes. These cosmic monsters gobble up matter and create vast swathes of space-time in their innards. It became clear that entanglement can’t account for all that virgin space-time. “The numerical value of the entanglement is not big enough to explain what happens behind black holes’ horizons,” says Susskind.
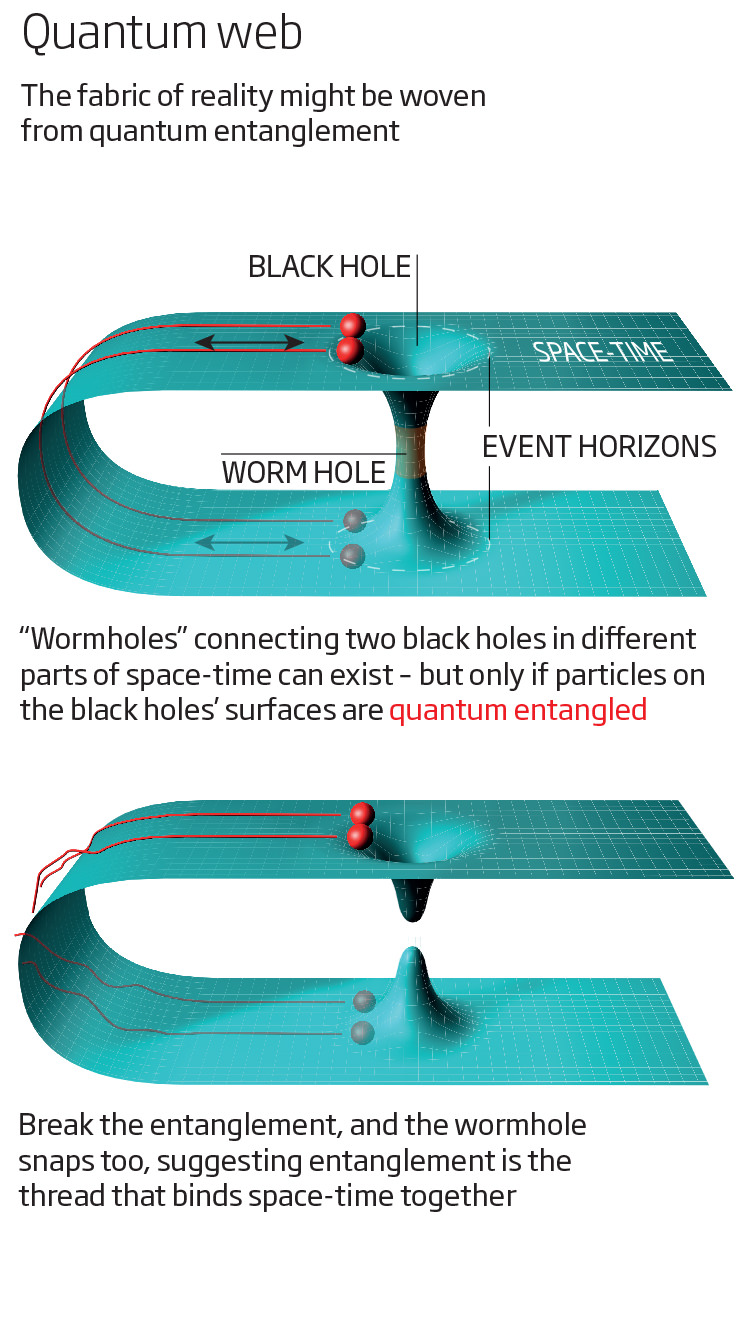
That needn’t be game over though. “There is another element, the capacity for complexity, which goes way beyond the capacity for entanglement,” says Susskind. Quantum systems can exist in a superposition of many different states at once, and complexity is a property that grows exponentially with the number of states. It’s the growth of black holes’ quantum complexity that Susskind reckons might be connected to the growth of space-time inside them, though he doesn’t yet have a clear sense of how that would work.
There is, however, a more fundamental objection to all this. The suggestion that entanglement can create space-time was spawned initially by the Maldacena duality, but its underlying mathematics still require a form of space-time to already exist. For Sean Carroll of the California Institute of Technology in Pasadena, the fact that space-time is not being created from scratch makes it problematic to say these quantum phenomena somehow underlie it. He too thinks there might be some fundamental link, and he’s exploring what that might be. But he is not using the duality to do it. “That would be cheating,” he says.
Carroll and his colleagues are taking a different tack. They have left out the confusing entity that is time for the moment (see “One time or another: Our best 5 theories of the fourth dimension“) and begun their work from an abstract mathematical object called a Hilbert space. The elements of this entity represent all the possible states of a quantum system. Any Hilbert space can be thought of as being built up from smaller such spaces. Carroll and his team looked at various Hilbert spaces, identified the smaller constituent spaces each was made from and figured out how much entanglement existed between them. Next, they tried to draw a graph in which the more entangled the parts, the closer they are together. Their question: does such a graph approximate to the familiar, smooth geometry of space?
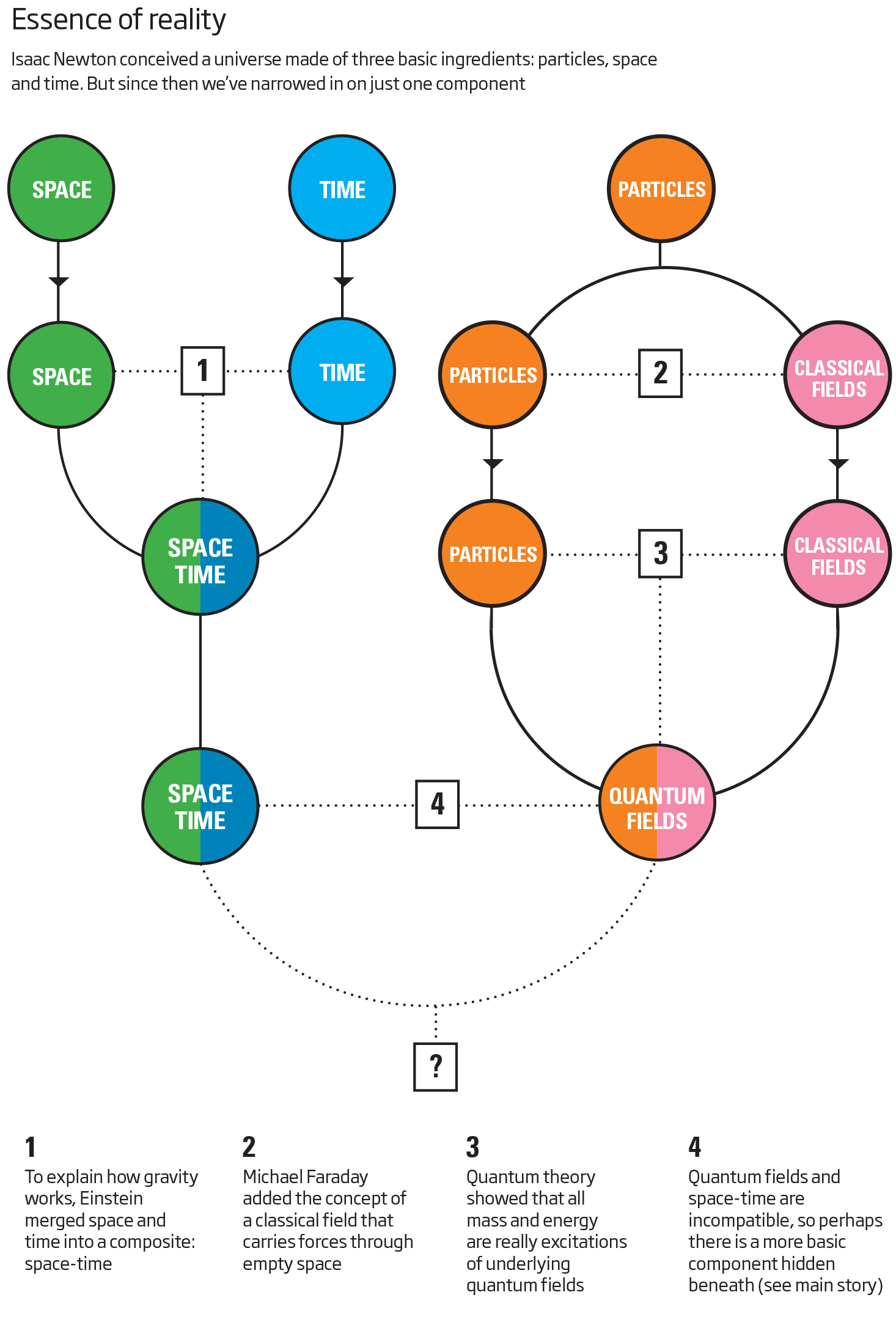
For any old graph, the answer was no. “It’ll be a horrible mess,” says Carroll. But when his team sifted through carefully, they did find some graphs that were promising. “There are very specific quantum states that look geometric, and those are the ones we are looking at,” says Carroll. For these, as one moved from one position on the graph to another, the transition was relatively smooth, suggesting the smooth geometry of space as we observe it could emerge from a purely quantum system.
“Saying space and time are made of entanglement only gets us so far”
But both Carroll’s and Maldacena’s lines of attack only take us so far. It is all very well to suggest that space and time are made of quantum entanglement and possibly quantum complexity – but what are they made of? Here is where we edge closer to finding the true bedrock of reality. Because both approaches suggest the same tantalising answer: information.
The mathematician and engineer Claude Shannon gave us a neat way to define information in 1948. He showed that the amount of information in something like a stream of bits or letters is related to its entropy, a measure of disorder. The greater the entropy, the greater the information. For example, a stream of three-bit numbers that are always 000 contains less information than a stream in which the numbers can also be 001, 101 or 111.
So in what sense is information at the root of things? Well, entanglement is information: the greater the entanglement between two systems, the more information they share. But there’s a caveat. The information Shannon defined certainly seems to exist and has real effects. Experiments just last year showed a nanomachine could use information to chill metal. But the sort of quantum information that might underlie space-time must be a little different. The information in a stream of words is about something. By contrast, the quantum information from which space emerges in Carroll’s work is just there. “The quantum state is not of or about anything,” says Carroll. “It is simply our best mathematical description of the universe.”
It actually makes sense that quantum information would be the foundation everything is built on, says Carroll. If you start with quantum mechanics and don’t presume anything else exists, then “basically all you have to play with is quantum information”. That would make information a basic constituent of the universe. “You can find people who think that information is all there is,” says Carroll.
We may even be able to test these ideas, says Sabine Hossenfelder of the Frankfurt Institute for Advanced Studies in Germany. “It’s exceedingly unlikely that something truly perfect ever comes into being,” she says. Just look at some of the most perfect structures we know of: crystals, defined as being composed of precisely repeating molecular units. In real life, even the most pristine crystals have defects. Something similar might happen if space-time is the product of something more basic. “If space-time is not fundamentally the real deal, then there should be defects left in it,” says Hossenfelder. The defects would lead to fleeting deviations from general relativity that we might be able to spot – by monitoring the behaviour of light arriving from billions of light years away, for example.
“There is no boundary to the universe, so the result could still be wrong”
We are a long way from tests like that. And, regardless, we still harbour a nagging doubt about the finding that started it all: the Maldacena duality.
The thing is, the sort of space Maldacena considered is not quite like real space. He was working with something called anti-de Sitter space, which is mathematically simpler. It has a well defined boundary and volume, the very things Maldacena was able to relate to one another.
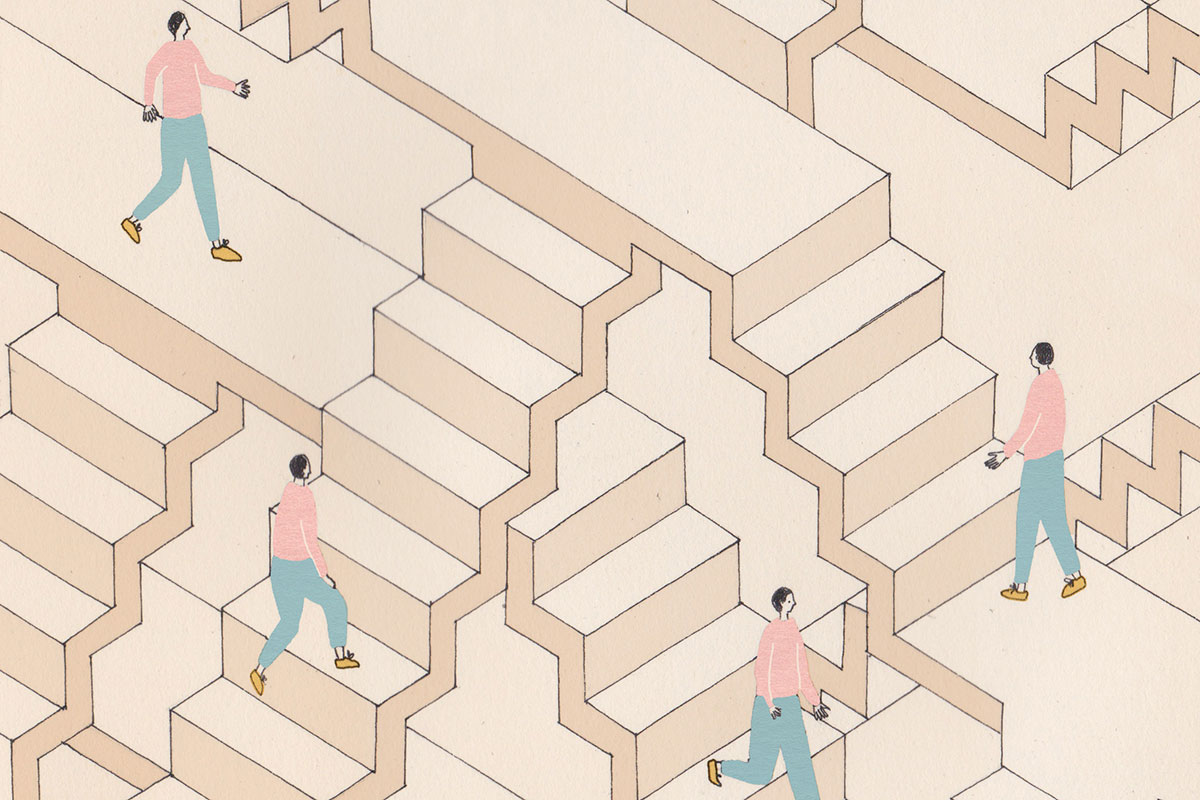
Harriet Lee-Merrion
It might sound uncontroversial, but the space we observe in the universe has important differences. Crucially, real space is expanding at an ever-increasing rate. This means that there is no sure boundary to our universe, and so no one is yet clear if the duality holds for real space. If it does not, that throws into question all the results built on top of it.
But although our universe does not have a boundary, it does have a horizon from beyond which nothing, not even light, can reach us. And this might offer a way forward. Last November, Erik Verlinde of the University of Amsterdam used this horizon and a set of assumptions to suggest that entanglement really is related to the space-time of our universe. “It’s motivated by some of the arguments that Susskind and Maldacena had in their picture,” Verlinde says. Based on this leap, he alighted on a modified form of Einstein’s equations and showed how space-time and gravity can emerge from entanglement even in ordinary space.
Though there’s considerable debate over some of Verlinde’s assumptions, there is excitement too. His work provides an explanation for dark energy, one of the most perplexing puzzles in physics. It is thought to be the driving force behind the expansion of space, but this effect arises naturally in Verlinde’s framework without the need for any mystery dark ingredient.
If Verlinde is correct, then maybe information really does underlie space and time in the real world. It would be a world in which every tangible aspect of reality is an illusion, with something ephemeral at the bottom.
But to Susskind at least, the idea that reality might be rooted in 0s and 1s is poetically beautiful. Perhaps, he says, we will one day be able to sum up the universe in a simple epigram: “ah, everything is information”.
No comments:
Post a Comment